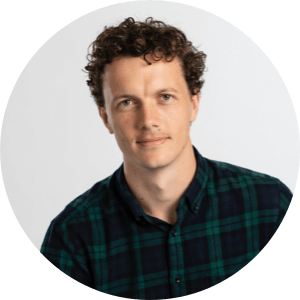
Dr Rohan Bythell-Douglas
Research Officer
Genome Stability Laboratory
Targeting telomere synthesis to kill ALT cancers
Cancers known as sarcomas form in tissues such as muscle, fat, nerves and tendons that connect, support and surround the body’s organs and bones. There are currently very poor survival rates for people diagnosed with this form of cancer.
Cells have an inbuilt mechanism, the telomeres, that determine how many times the cell can replicate. Telomeres shorten each time the cell divides to reproduce itself – eventually reaching a critically short length that prevents further division.
Cancer cells evade this normal process by finding ways to extend their telomeres. Sarcomas and other cancers use a mechanism called “ALT”, in which DNA is added to the end of the telomere by copying it from somewhere else. The ALT process is active in 45 to 60% of sarcoma tumours.
Treatment options for patients with sarcoma have not changed in the past 35 years, in part because no suitable ALT specific drug target has been identified.
Dr Rohan Bythell-Douglas and his colleagues have identified a key weakness in the ALT pathway. Targeting this weakness not only stops telomeres from being extended, it specifically and quickly kills the cancer cells.
The team has also identified drug fragments that target the ALT weakness. While these drug fragments don’t yet present a treatment option, Rohan’s project will use X-ray crystallography to see how the fragments could be modified to improve their effect.
“We aim to build an informed strategy for modifying the drug fragments into more mature drug-like molecules, with the goal of developing a drug which could be used in the clinic to treat sarcomas,” says Rohan.
Rohan is an expert in the biochemistry and structural biology of DNA repair enzymes involved in cancer and rare genetic diseases. His goal is to uncover the key mechanistic details of DNA damage repair, down to the resolution of individual amino acids. This level of detail helps Rohan and his colleagues understand how genetic mutations in individual people can increase their risk of disease, and supports the design of new drug treatments.
Rohan’s Rising Star project brings together the components of his research career to date: biochemistry, structural biology and digital protein modelling.

Dr Jacki Heraud-Farlow
Research Officer
Cancer & RNA Biology Laboratory
Investigating new targets to treat rare childhood autoinflammatory disease
Aicardi-Goutières Syndrome (AGS) is a rare autoinflammatory disease which starts in early childhood. The disease causes profound degeneration in the brain and a loss of motor and communication skills. There are currently no targeted therapies available. A particular characteristic of AGS is the inappropriate production of a powerful signalling molecule, called interferon. Essential to the ability of our immune system to respond to viruses and bacteria, interferon can be highly damaging to the body in the absence of infection.
By investigating the molecules and genes that lead to the development of AGS, we hope to find new therapies to treat this devastating disease. Understanding this rare condition can also point to new treatment opportunities that harness the immune system to treat common conditions like cancer, or to fight infection by RNA viruses (like COVID-19).
Dr Jacki Heraud-Farlow has identified never-before-seen regulators of inflammation in mice that have been genetically modified to develop a condition that mimics AGS. These regulators represent a new treatment target that could stop the inappropriate production of interferon that is so damaging in people with this disease.
Jacki’s collaboration with a clinician at Murdoch Children’s Research Institute who treats AGS patients will allow her to collect blood samples from people with the disease. She will use these cells to generate human cell lines, enabling Jacki to further study the new regulators she has discovered and test their ability to modify the interferon response.
“If we prove successful, this would be a highly significant advance in the development of new treatments for AGS. In addition, the human cell lines generated from patient samples will provide an invaluable tool for future testing of other potential therapies,” says Jacki.
Jacki is an early/mid-career researcher who specialises in RNA biology. She is particularly interested in how RNA structures and modifications shape the balance between immunity to viruses and autoimmunity against our cells’ own RNA, and how this can be manipulated to develop novel therapies to treat rare and common diseases.
Originally from Melbourne, Jacki completed her PhD at the University of Vienna in a specialised RNA biology program, studying RNA regulation in neurons. She joined SVI’s Cancer and RNA biology lab, led by Professor Carl Walkley, in 2016 and is now a Team Leader within the group, studying AGS and RNA editing.
Dr Natalie Wee
Research Officer
Bone Cell Biology & Disease Unit
Defining the signals that stimulate bone formation where it is needed most
It starts with a fracture: a broken bone that should easily mend, given time. But when the break is caused by osteoporosis, the consequences can be devastating.
Osteoporosis affects over one million Australians. It weakens the skeleton, increasing the risk of fractures, particularly to the hip, spine, and wrist. Too often these injuries are the trigger for ongoing health issues that have huge personal and social costs. Alarmingly, people who suffer a fragility fracture, particularly to the hip, are at higher risk of dying, with death rates of up to 24% in the first year after the break.
Current therapies can preserve bone strength in the spine, but they don’t work on other areas, such as the femur, hip or wrist – the sites that need strength the most when someone falls.
The femur, hip and wrist bones are strong because they are rich in cortical bone, the hard-outer shell. It’s cortical bone strength that determines if someone will suffer a hip or wrist fracture, should they fall.
Finding a way to increase cortical bone width is key to helping those with osteoporosis – but to do that, we need to know what is happening at a cellular level.
Dr Wee is studying the unique cells on the outside of cortical bone, identifying which ones can make bones wider, and the signals they respond to. She is also investigating why bone width is different in men and women – females have narrower bones, meaning they are more likely to have osteoporosis.
Her work is an important first step towards designing therapies that can specifically strengthen the wrist, hip and femur of those with weak bones
Dr Natalie Wee’s PhD examined how an appetite-stimulating molecule influenced bone mass during diet-induced obesity and mild cold stress. This work cut across bone biology, neuroscience and energy metabolism, and allowed her to develop new approaches to studying the skeleton.
After completing her PhD, Dr Wee travelled to the USA for further training. At Washington University in St Louis she developed techniques to quantify nerves in skeletal sites, and at the University of Connecticut Health Center she led several bone biology projects and learnt cutting-edge techniques for lineage tracing of bone cells.
Her work has been recognised through oral presentations at major conferences, national and international travel grants and numerous publications. She is also an active member of the scientific community, and has been involved in mentoring programs, teaching and serving on committees at institutional and national levels.
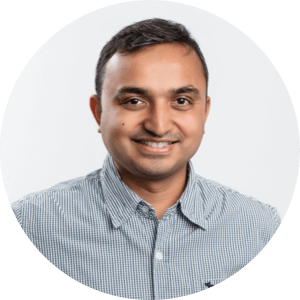
Dr Prerak Trivedi
Research Officer
Islet Biology Unit
Disarming immune cells to prevent type 1 diabetes
The job of the immune system is to protect us from viral and bacterial infections. However, sometimes this process goes awry and the immune system attacks normal cells, leading to the development of autoimmune disease.
In type 1 diabetes, immune cells mistakenly target the insulin-producing cells in the pancreas, called beta cells.
These immune cell are long-lived and retain their function over a long period of time. Even after a person has been diagnosed with type 1 diabetes, the immune cells continue to contribute to ongoing destruction of beta cells. To achieve prevention of type 1 diabetes, we need to find new ways of eliminating this immune attack.
Immune cells that recognise beta cells sometimes go into a state of hibernation, which may help them to elude current therapies. But even in hibernation, these immune cells need to generate energy for survival and to maintain their function.
Dr Prerak Trivedi aims to identify the energy pathways upon which immune cells rely, by using state-of-the-art technologies and in collaboration with experts in the field of metabolism (how cells produce and manage energy).
“By discovering the metabolic pathways that are used by the immune cells, I hope to identify new ways to ‘disarm’ them, preventing destruction of insulin-producing beta cells”, says Prerak.
Prerak is a molecular and cellular immunologist who works to understand the function of the immune system in health and disease. During his PhD studies in SVI’s Immunology and Diabetes Lab, he studied how immune cells recognise and kill beta cells. His work was key to initiating SVI’s BANDIT clinical trial for the treatment of type 1 diabetes. After completing his PhD, Prerak moved to New York’s Memorial Sloan Kettering Cancer Centre, researching immune cells in cancer. In 2021, Prerak returned to SVI to study the function and differentiation of immune cells in type 1 diabetes.
Prerak’s research has been recognised in publications, oral, poster presentations and fellowships/awards, as well as in the establishment of collaborations with academia and bio-pharma industries. His work in the scientific community includes mentoring students, peer-reviewing and participation in scientific societies and associations.
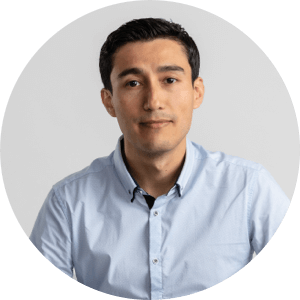
Dr Carlos Andres Peña Solorzano
Research Officer
Bioinformatics & Cellular Genomics Laboratory
Improving breast cancer diagnosis by applying artificial intelligence to mammograms
Population-based breast screening programs have been shown to increase the likelihood of diagnosing cancer in its early stages. However, typical wait times for results are two weeks or more – the time it takes for two expert reads of a mammogram, and a third if interpretations differ. Despite this thorough approach, in 2018 more than 34,000 Australian women (in the age-group 50 to 74 years) were recalled for assessment, and later determined not to have breast cancer.
Artificial Intelligence (AI) has the potential to reduce the workload of radiologists by filtering out normal mammograms. But AI’s performance on cancer diagnosis has been limited, because the highly detailed information required to adequately ‘train’ the digital ‘brain’ has to date been lacking.
As part of the Breast Cancer AI project (BRAIx) and with the help of BreastScreen Victoria, Dr Carlos Peña Solorzano is working with radiologists to build a manually annotated database that can be used to ‘teach’ and verify the performance of AI-based interpretation of mammograms. This database will include highly specific visual details like cancer location and the contours of cancer tissue, which human experts use to visually identify cancers in mammograms.
Carlos’ project will benchmark the use of this kind of database for the improvement of breast cancer diagnosis and localisation using AI on mammograms.
“Our results will inform the future development of free-to-use mammogram databases and provide a baseline for the wider medical research community developing automated tools for breast cancer diagnosis,” says Carlos.
Carlos is an expert in the use of AI for the processing of medical images, including CT (computed tomography), X-rays, and mammogram scans. His goal is to improve patient care through better use of AI in areas such as disease diagnosis, organ segmentation and automatic medical database labelling.
Carlos joined SVI’s Bioinformatics & Cellular Genomics Laboratory in 2020 as a postdoctoral researcher. He trained during his PhD to use AI for organ characterisation on post-mortem CT scan databases and has more than five years’ experience in the application of AI for image processing and process automation.